Streptococcal pyrogenic exotoxin B cleaves GSDMA and triggers pyroptosis - Nature.com
Abstract
Gasdermins, a family of five pore-forming proteins (GSDMA–GSDME) in humans expressed predominantly in the skin, mucosa and immune sentinel cells, are key executioners of inflammatory cell death (pyroptosis), which recruits immune cells to infection sites and promotes protective immunity1,2. Pore formation is triggered by gasdermin cleavage1,2. Although the proteases that activate GSDMB, C, D and E have been identified, how GSDMA—the dominant gasdermin in the skin—is activated, remains unknown. Streptococcus pyogenes, also known as group A Streptococcus (GAS), is a major skin pathogen that causes substantial morbidity and mortality worldwide3. Here we show that the GAS cysteine protease SpeB virulence factor triggers keratinocyte pyroptosis by cleaving GSDMA after Gln246, unleashing an active N-terminal fragment that triggers pyroptosis. Gsdma1 genetic deficiency blunts mouse immune responses to GAS, resulting in uncontrolled bacterial dissemination and death. GSDMA acts as both a sensor and substrate of GAS SpeB and as an effector to trigger pyroptosis, adding a simple one-molecule mechanism for host recognition and control of virulence of a dangerous microbial pathogen.
This is a preview of subscription content
Access options
Subscribe to Journal
Get full journal access for 1 year
199,00 €
only 3,90 € per issue
Tax calculation will be finalised during checkout.
Buy article
Get time limited or full article access on ReadCube.
$32.00
All prices are NET prices.
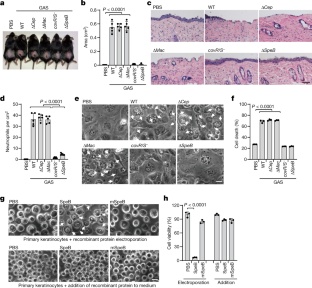
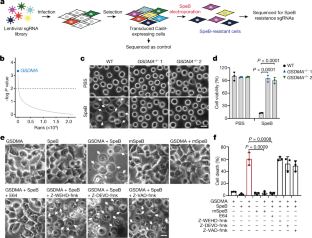
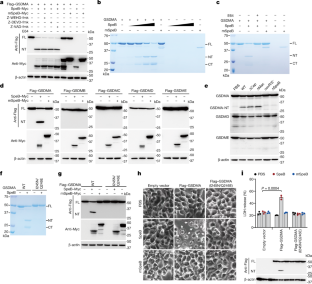
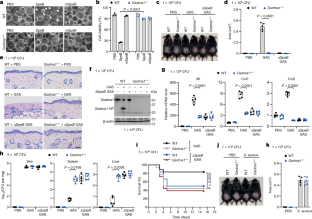
Data availability
All data supporting the findings of this study are included in this manuscript and its supplementary information. Source data are provided with this paper.
References
Broz, P., Pelegrin, P. & Shao, F. The gasdermins, a protein family executing cell death and inflammation. Nat. Rev. Immunol. 20, 143–157 (2020).
Liu, X., Xia, S., Zhang, Z., Wu, H. & Lieberman, J. Channelling inflammation: gasdermins in physiology and disease. Nat. Rev. Drug Discov. 20, 384–405 (2021).
Cole, J. N., Barnett, T. C., Nizet, V. & Walker, M. J. Molecular insight into invasive group A streptococcal disease. Nat. Rev. Microbiol. 9, 724–736 (2011).
Musser, J. M., Stockbauer, K., Kapur, V. & Rudgers, G. W. Substitution of cysteine 192 in a highly conserved Streptococcus pyogenes extracellular cysteine protease (interleukin 1beta convertase) alters proteolytic activity and ablates zymogen processing. Infect. Immun. 64, 1913–1917 (1996).
Svensson, M. D. et al. Role for a secreted cysteine proteinase in the establishment of host tissue tropism by group A streptococci. Mol. Microbiol. 38, 242–253 (2000).
Cole, J. N. et al. Trigger for group A streptococcal M1T1 invasive disease. FASEB J. 20, 1745–1747 (2006).
Shelburne, S. A., 3rd et al. Growth characteristics of and virulence factor production by group A Streptococcus during cultivation in human saliva. Infect. Immun. 73, 4723–4731 (2005).
Aziz, R. K. et al. Invasive M1T1 group A Streptococcus undergoes a phase-shift in vivo to prevent proteolytic degradation of multiple virulence factors by SpeB. Mol. Microbiol. 51, 123–134 (2004).
Liu, X. et al. Inflammasome-activated gasdermin D causes pyroptosis by forming membrane pores. Nature 535, 153–158 (2016).
Ding, J. et al. Pore-forming activity and structural autoinhibition of the gasdermin family. Nature 535, 111–116 (2016).
Aglietti, R. A. et al. GsdmD p30 elicited by caspase-11 during pyroptosis forms pores in membranes. Proc. Natl Acad. Sci. USA 113, 7858–7863 (2016).
Sborgi, L. et al. GSDMD membrane pore formation constitutes the mechanism of pyroptotic cell death. EMBO J. 35, 1766–1778 (2016).
Sumitomo, T. et al. Streptococcal cysteine protease-mediated cleavage of desmogleins is involved in the pathogenesis of cutaneous infection. Front. Cell Infect. Microbiol. 8, 10 (2018).
Saouda, M., Wu, W., Conran, P. & Boyle, M. D. Streptococcal pyrogenic exotoxin B enhances tissue damage initiated by other Streptococcus pyogenes products. J. Infect. Dis. 184, 723–731 (2001).
Paz, I. et al. Galectin-3, a marker for vacuole lysis by invasive pathogens. Cell Microbiol. 12, 530–544 (2010).
Doran, J. D. et al. Autocatalytic processing of the streptococcal cysteine protease zymogen: processing mechanism and characterization of the autoproteolytic cleavage sites. Eur. J. Biochem. 263, 145–151 (1999).
Gerwin, B. I., Stein, W. H. & Moore, S. On the specificity of streptococcal proteinase. J. Biol. Chem. 241, 3331–3339 (1966).
Nomizu, M. et al. Substrate specificity of the streptococcal cysteine protease. J. Biol. Chem. 276, 44551–44556 (2001).
Carroll, R. K. & Musser, J. M. From transcription to activation: how group A Streptococcus, the flesh-eating pathogen, regulates SpeB cysteine protease production. Mol. Microbiol. 81, 588–601 (2011).
Lichtenberger, B. M. et al. Epidermal EGFR controls cutaneous host defense and prevents inflammation. Sci. Transl. Med. 5, 199ra111 (2013).
Sawada, Y. et al. Cutaneous innate immune tolerance is mediated by epigenetic control of MAP2K3 by HDAC8/9. Sci. Immunol. 6, eabe1935 (2021).
Kolaczkowska, E. & Kubes, P. Neutrophil recruitment and function in health and inflammation. Nat. Rev. Immunol. 13, 159–175 (2013).
Barnett, T. C. et al. The globally disseminated M1T1 clone of group A Streptococcus evades autophagy for intracellular replication. Cell Host Microbe 14, 675–682 (2013).
LaRock, C. N. et al. IL-1β is an innate immune sensor of microbial proteolysis. Sci. Immunol. 1, eaah3539 (2016).
Nitsche-Schmitz, D. P., Rohde, M. & Chhatwal, G. S. Invasion mechanisms of Gram-positive pathogenic cocci. Thromb. Haemost. 98, 488–496 (2007).
Rohde, M. & Chhatwal, G. S. Adherence and invasion of streptococci to eukaryotic cells and their role in disease pathogenesis. Curr. Top. Microbiol. Immunol. 368, 83–110 (2013).
Hynes, W. & Sloan, M. in Streptococcus pyogenes: Basic Biology to Clinical Manifestations (eds Ferretti, J. J. et al.) (2016).
Zhang, W., Rong, C., Chen, C. & Gao, G. F. Type-IVC secretion system: a novel subclass of type IV secretion system (T4SS) common existing in gram-positive genus Streptococcus. PLoS ONE 7, e46390 (2012).
Richter, J. et al. Streptolysins are the primary inflammasome activators in macrophages during Streptococcus pyogenes infection. Immunol. Cell Biol. 99, 1040–1052(2021).
Richter, J., Brouwer, S., Schroder, K. & Walker, M. J. Inflammasome activation and IL-1β signalling in group A Streptococcus disease. Cell Microbiol. 23, e13373 (2021).
Brouwer, S., Barnett, T. C., Rivera-Hernandez, T., Rohde, M. & Walker, M. J. Streptococcus pyogenes adhesion and colonization. FEBS Lett. 590, 3739–3757 (2016).
Carothers, K. E. et al. The streptococcal protease SpeB antagonizes the biofilms of the human pathogen Staphylococcus aureus USA300 through cleavage of the staphylococcal SdrC protein. J. Bacteriol. 202, e00008-e00020 (2020).
Zhou, W. et al. Structure of the human cGAS–DNA complex reveals enhanced control of immune surveillance. Cell 174, 300–311.e311, (2018).
Kranzusch, P. J. et al. Ancient origin of cGAS–STING reveals mechanism of universal 2′,3′ cGAMP signaling. Mol. Cell 59, 891–903 (2015).
Orzalli, M. H. & Kagan, J. C. A one-protein signaling pathway in the innate immune system. Sci. Immunol. 1, eaah6184 (2016).
Mitchell, P. S., Sandstrom, A. & Vance, R. E. The NLRP1 inflammasome: new mechanistic insights and unresolved mysteries. Curr. Opin. Immunol. 60, 37–45 (2019).
Whatmore, A. M. & Kehoe, M. A. Horizontal gene transfer in the evolution of group A streptococcal emm-like genes: gene mosaics and variation in Vir regulons. Mol. Microbiol. 11, 363–374 (1994).
Zheng, Z. Z. et al. The lysosomal Rag–Ragulator complex licenses RIPK1-and caspase-8-mediated pyroptosis by Yersinia. Science 372, eabg0269(2021).
Li, W. et al. Quality control, modeling, and visualization of CRISPR screens with MAGeCK-VISPR. Genome Biol. 16, 281, (2015).
Shepard, L. A., Shatursky, O., Johnson, A. E. & Tweten, R. K. The mechanism of pore assembly for a cholesterol-dependent cytolysin: formation of a large prepore complex precedes the insertion of the transmembrane β-hairpins. Biochemistry 39, 10284–10293 (2000).
Acknowledgements
We thank V. Nizet for sharing S. pyogenes isolate M1T1 strain 5448 and its isogenic mutant strains (ΔspeB, covR/S−, ΔcepA and Δmac variants) as well as SpeB constructs, Z. Zhang for providing S. pyogenes M9, M12 amd M73 strains, C. Wang for providing Phage-Flag vector, and Y. Chen for providing a modified pET vector with an N-terminal 6×His-SUMO tag. This work was supported by National Key R&D Program of China (2020YFA0509600), National Natural Science Foundation of China (32122034, 31972897), Key Research Program of the Chinese Academy of Sciences (ZDBS-LY-SM008), Shanghai Pilot Program for Basic Research–Chinese Academy of Sciences, Shanghai Branch (JCYJ-SHFY-2021-009), Strategic Priority Research Program of Chinese Academy of Sciences (XDB29030300), Shanghai Municipal Science and Technology Major Project (2019SHZDZX02) (X.L.), NIH R01CA240955 and R01AI39914 (J.L.), NIH R01AI127654 (T.S.K.) and China Postdoctoral Science Foundation (2019M650193), Guangzhou Science and Technology Project (202102020093) (W.D.).