Both chimpanzee adenovirus-vectored and DNA vaccines induced long-term immunity against Nipah virus infection ... - Nature.com
Abstract
Nipah virus (NiV) is a highly lethal zoonotic paramyxovirus that poses a severe threat to humans due to its high morbidity and the lack of viable countermeasures. Vaccines are the most crucial defense against NiV infections. Here, a recombinant chimpanzee adenovirus-based vaccine (AdC68-G) and a DNA vaccine (DNA-G) were developed by expressing the codon-optimized full-length glycoprotein (G) of NiV. Strong and sustained neutralizing antibody production, accompanied by an effective T-cell response, was induced in BALB/c mice by intranasal or intramuscular administration of one or two doses of AdC68-G, as well as by priming with DNA-G and boosting with intramuscularly administered AdC68-G. Importantly, the neutralizing antibody titers were maintained for up to 68 weeks in the mice that received intramuscularly administered AdC68-G and the prime DNA-G/boost AdC68-G regimen, without a significant decline. Additionally, Syrian golden hamsters immunized with AdC68-G and DNA-G via homologous or heterologous prime/boost immunization were completely protected against a lethal NiV virus challenge, without any apparent weight loss, clinical signs, or pathological tissue damage. There was a significant reduction in but not a complete absence of the viral load and number of infectious particles in the lungs and spleen tissue following NiV challenge. These findings suggest that the AdC68-G and DNA-G vaccines against NiV infection are promising candidates for further development.
Similar content being viewed by others
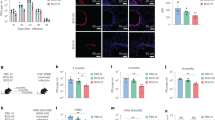
BCG immunization induces CX3CR1hi effector memory T cells to provide cross-protection via IFN-γ-mediated trained immunity
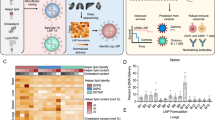
Nanoparticle-based DNA vaccine protects against SARS-CoV-2 variants in female preclinical models
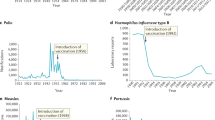
A guide to vaccinology: from basic principles to new developments
Introduction
Nipah virus (NiV), a member of the Henipavirus genus in the Paramyxoviridae family, is an enveloped, negative-sense, single-stranded RNA virus. The first recorded cases of NiV occurred in 1998 during an outbreak of severe encephalitis in pig farmers from Malaysia and abattoir workers in Singapore, with a 39.6% case fatality rate (CFR)1,2,3. It was later discovered that pigs were most likely infected after eating fruit contaminated by infected fruit bats of the genus Pteropus4,5. Humans can become infected with NiV through exposure to sick pigs, but there have been no documented cases of human-to-human transmission6. Since the initial outbreak in Malaysia, NiV outbreaks have occurred almost annually in Bangladesh and India between 2001 and 20187,8. NiV can spread directly from bats to humans through the consumption of contaminated raw date palm sap in Bangladesh9, with a higher fatality rate ranging from 40% to 75%10, and human-to-human transmission has been observed in these outbreaks11,12. The latest outbreaks occurred in 2021 in Kerala, India, in which a 12-year-old boy died due to NiV infection13. Based on zoonotic spillover events and genetic analysis, at least two distinct major strains, NiV-Malaysia (NiV-M) and NiV-Bangladesh (NiV-B), have been implicated in previous outbreaks in humans14,15 Although there are only approximately 650 cases of NiV infection in these outbreaks, the mortality rate can be as high as 100% depending on the context and limitations of the health-care systems in which outbreaks occur.
The symptoms of NiV disease (NVD) include fever, headache, cough, sore throat, difficulty breathing, and vomiting. The illness can progress to more severe symptoms, such as disorientation, drowsiness, acute respiratory illness, and fatal encephalitis16,17. Most people who survive acute encephalitis fully recover, but long-term side effects of Nipah virus infection have been reported in survivors, including persistent convulsions and personality changes10,18.
Unlike other paramyxoviruses, NiV exhibits a broad host range, including humans, pigs, dogs, cats, horses, guinea pigs, hamsters, and fruit bats19,20. NiV uses the highly conserved ephrinB2 and ephrinB3 as host cell entry receptors, which explains its preferential tropism for the lungs, brain, and spleen, all of which express high levels of these cellular receptors21,22,23.
NiV is a risk group 4 pathogen due to its pandemic potential, extreme pathogenicity, and lack of medical treatments. It is listed as a priority pathogen by the World Health Organization (WHO)24, but currently, no licensed vaccine or antiviral drug is available. The current NiV vaccine development platform is based on NiV glycoprotein (G) and/or fusion protein (F) and aims to induce the production of NiV neutralizing antibodies. Multiple vaccine approaches have been tested in animal models and have been shown to be effective, such as a recombinant adeno-associated virus vaccine expressing NiV-M G completely protecting hamsters against the NiV-M challenge25, a recombinant VSV-vectored vaccine expressing NiV-B G protecting African green monkeys against a homologous NiV-B challenge26, and a canarypox virus-based vaccine vector expressing NiV-M G or F protecting against NiV-M in pigs27. In addition, a messenger RNA (mRNA-1215) vaccine encoding NiV-M F and G was tested in phase I clinical trials in 202228. Additionally, researchers are also exploring other vaccine platforms to develop novel NiV vaccines with better immune protection effects.
Several vaccines based on AdC68 vectors, including the Ebola virus (EBOV), Middle East respiratory syndrome coronavirus (MERS-CoV), and severe acute respiratory syndrome coronavirus 2 (SARS‑CoV‑2) vaccines, have been developed due to low pre-existing immunity in humans29, their broad tissue tropism, their inability to replicate after immunization, and their ability to induce strong and long-lasting immunity30,31,32. In addition, DNA-based vaccines have several advantages, including rapid production, easy design, low temperature sensitivity, and affordability, which are critical elements for outbreak areas. Here, we developed two vaccines, a DNA vaccine (DNA-G) and a recombinant chimpanzee adenovirus vaccine (AdC68-G), both of which express NiV-G, and their immunogenicity and efficacy were tested in mice and hamsters. The results show that the two vaccines induced the production of long-lasting neutralizing antibodies and robust T-cell responses against the G protein after vaccination in BALB/c mice, as well protection from lethal NiV-M and NiV-B infection challenge in Syrian golden hamsters.
Results
AdC68-G and DNA-G were successfully constructed and characterized
The consensus G protein of NiV shown in Supplementary Fig. 1a was selected as the antigen for vaccine development. The results of phylogenetic tree analysis among the sequence in the study and other unduplicated and complete G protein sequences in GenBank were shown in Supplementary Fig. 1b. The acquired G amino acid sequence in the study has homology with the challenged Malaysian and Bangladesh NiV strains of 96.2% and 99.5% respectively. To develop a recombinant chimpanzee adenovirus-based vaccine (AdC68-G), the full-length G sequence was inserted into the E1-deleted region (E1) of the adenoviral vector (AdC68) and placed under the control of the cytomegalovirus (CMV) promoter (Fig. 1a). In addition, the G gene was subcloned into the mammalian expression vector pVAX1 to generate DNA-G (Fig. 1b). Transfection of HEK293 cells with the linearized recombinant plasmid pAdC68-G resulted in cytopathic effects 8–10 days later (Fig. 1c). The expression of the G protein was evaluated by Western blot analysis of HEK293 cells infected with AdC68-G and transfected with DNA-G using mouse anti-NiV G serum. The results showed high expression of the G protein by AdC68-G and DNA-G, while no G-specific band was detected in the control group (Fig. 1d, e), demonstrating that both a recombinant chimpanzee adenovirus-based vaccine and a DNA-based vaccine that express the full-length codon-optimized NiV G protein were successfully constructed.
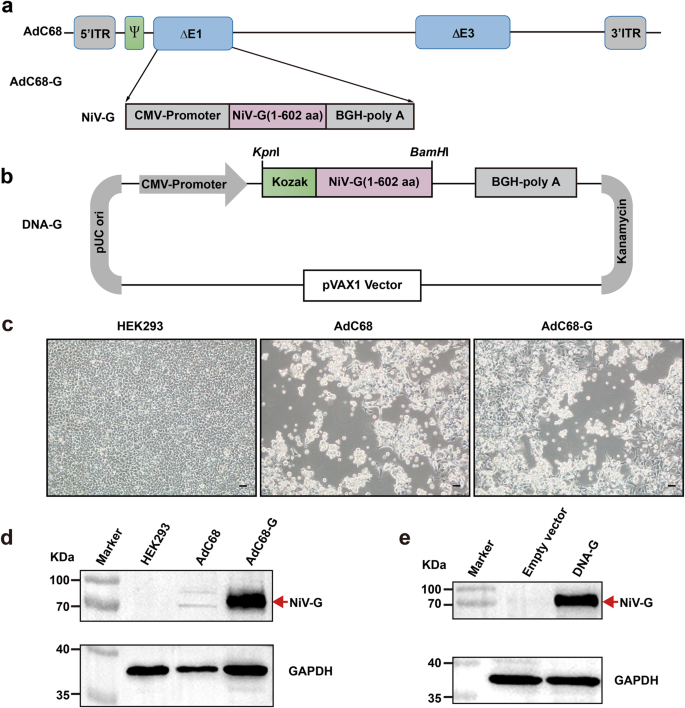
a Schematic diagram of the genome of AdC68-G and the coding sequence for the NiV G protein. b Diagram of plasmid DNA-G. The plasmid pVAX1 vector was used to engineer a gene cassette containing a CMV promoter, the Kozak sequence, NiV-G cDNA, and a BGH pA signal element. c The cytopathic effect (CPE) was observed in HEK293 cells transfected with the linearized AdC68-G or AdC68 plasmid. d Western blot analysis of HEK293 cells infected with rescued recombinant AdC68-G or AdC68 viruses. e Western blot analysis of HEK293T cells transfected with DNA-G (pVAX1-G) or empty vector (pVAX1). GAPDH was used as the internal control. All blots derive from the same experiment and that they were processed in parallel. Bar scale, 100 µm.
Immunization with AdC68-G alone or in combination with DNA-G vaccine induce robust cellular robust cellular and sustained humoral immune responses in BALB/c mice
To evaluate the immunogenicity of the two vaccines, female BALB/c mice aged 6-8 weeks in ten groups were immunized in two batches (Fig. 2a). The first batch (groups 1 to 4) and the second batch (groups 5–10) were followed for 29 and 68 weeks, respectively. In groups 2 and 4, 5 × 1010 viral particles (VPs) of AdC68-G were administered to mice in one dose or two doses at a 21-day interval via the intranasal (i.n.) route, while mice in groups 1 and 3 received 5 × 1010 VPs of AdC68-empty vector and were used as controls. In groups 6 and 8, 5 × 1010 VPs of AdC68-G was administered to mice in one dose or two doses at a 21-day interval via intramuscular (i.m.) injection, while mice in groups 5 and 7 received 5 × 1010 VPs of AdC68 vector and were used as controls. Mice in group 10 received a heterologous DNA-G (50 μg) prime/AdC68-G (5 × 1010 VPs) boost vaccine regimen with a 3-week interval, while mice in group 9 were given the corresponding empty vector as a control (Fig. 2b).
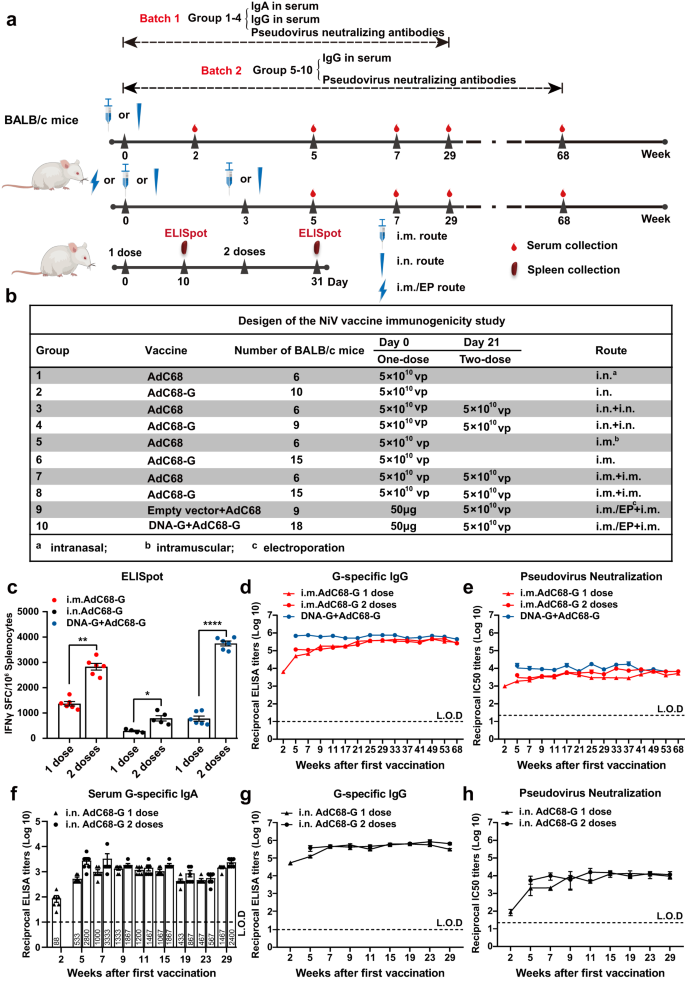
Experimental scheme. BALB/c mice were immunized with a recombinant vaccine (AdC68-G or DNA-G) either once (1 dose) or twice (2 doses) at a 21-day interval (a) with the indicated schedule, dosage, and route (b). Ten days after each immunization, splenocytes were harvested for NiV-specific T-cell response analysis (a, lower). c T-cell responses induced by the vaccine regimens. IFN-γ-secreting splenocytes induced by the vaccine in BALB/c mice were detected by ELISpot at 10 days after the first or second immunization. Splenic lymphocytes were stimulated with two peptide pools corresponding to the NiV-G region. The IgG antibody (d) and pseudovirus neutralization antibody (e) responses in the sera of mice immunized with AdC68-G either once (1 dose) or twice (2 doses) via the i.m. route and the DNA-G prime/AdC68-G boost immunization regimen. The IgA antibodies (f), IgG antibodies (g) and pseudovirus neutralization antibodies (h) in the sera of mice immunized with AdC68-G either once (1 dose) or twice (2 doses) via the i.n. route. Data are shown as the mean ± SEM. Two-tailed unpaired Student's t tests were conducted to compare differences between two experimental groups *p < 0.05, **p < 0.01, ****p < 0.0001. Error bars indicate 95% confidence intervals. L.O.D. represents the limit of detection.
To detect NiV-specific T-cell responses, splenocytes were harvested for analysis of the ability to produce interferon (IFN)-γ 10 days after vaccination. The results showed that a strong cellular immune response was induced after one dose of vaccine, while a higher level of IFN-γ produced by T cells was detected after two doses of vaccine (p < 0.01, p < 0.05 and p < 0.0001 in the three different groups). After two doses, the DNA-G prime/AdC68-G boost vaccine group exhibited the highest level of IFN-γ among all regimen groups, with an average of 3746 spot-forming units (SFU) per 106 splenocytes (Fig. 2c).
Serum samples were collected up to 68 weeks post-vaccination in groups 5 to 10, and humoral immune responses with the vaccine regimens were evaluated by enzyme-linked immunosorbent assays (ELISA) and pseudovirus neutralization assay using an HIV-luciferase system. A single i.m. injection of the AdC68-G vaccine was able to induce IgG antibody titers of 105. After homologous boosting with the AdC68-G vaccine, IgG antibody titers were slightly elevated (p < 0.01). Heterologous immunization with the DNA-G prime/AdC68-G boost regimen achieved the highest IgG antibody titers, with an average titer of 443733 at 68 weeks after two doses (p < 0.0001). Notably, the serum IgG antibody levels of mice in all immunized groups did not decrease significantly until 68 weeks after immunization (Fig. 2d). In accordance with the IgG antibody results, neutralization antibody titers exhibited the same results. The highest average IC50 of pseudovirus-neutralizing antibodies (pNAbs) in 68-week immune sera was 6729 for the DNA-G prime/AdC68-G boost immunization group (Fig. 2e).
The humoral immune responses induced by AdC68-G vaccine via i.n. immunization was investigated in mice. G-specific IgA and IgG antibodies were detected as early as two weeks after the first vaccination, and their titers increased upon boosting with AdC68-G (p < 0.01) (Fig. 2f, g). In addition, pNAbs whose production was induced by i.n immunization were more potent than those induced by i.m. immunization (Fig. 2g, h). The average IC50 of pNAbs in 29-week immune sera was 9303 for the single-dose AdC68-G (i.n.) group and 11,570 for the two-dose AdC68-G (i.n.) group (Fig. 2h).
These findings suggested that all vaccine regimens elicited effective cellular and long-term humoral immune responses in BALB/c mice. However, the two-dose immunization groups exhibited stronger cellular and higher-level humoral immune responses than the single-dose groups. I.n. immunization with AdC68-G induced a stronger humoral immune response than i.m. immunization, and the heterologous DNA-G prime/AdC68-G boost regimen induced a higher humoral and cellular immune response than the homologous AdC68-G/AdC68-G i.m. immunization protocols.
AdC68-G and DNA-G vaccines elicited the production of virus-neutralizing antibodies against two different strains of NiV in Syrian golden hamsters
Before determining whether the AdC68-G and DNA-G vaccines provide protection against NiV infection, the immune response induced by these vaccine regimens was evaluated in hamsters. Based on the immunogenicity of vaccination in BALB/c mice, a two-dose regimen was chosen for this study, with the addition of a three-dose DNA-G vaccine regimen. The vaccination schedule of the study is summarized in Fig. 3a. Briefly, hamsters were vaccinated on day 0 with AdC68-G (i.n. or i.m.) or DNA-G intramuscularly and electroporation (i.m./EP) and boosted with one dose of AdC68-G (i.n. or i.m.) or two doses of DNA-G (i.m./EP) at 21-day intervals. As a negative control, hamsters were vaccinated with empty AdC68 or pVAX1 vectors at the same time. Blood samples were collected at 3 weeks after each vaccination. High levels of G-specific IgG and pNAbs were detected after a single dose.
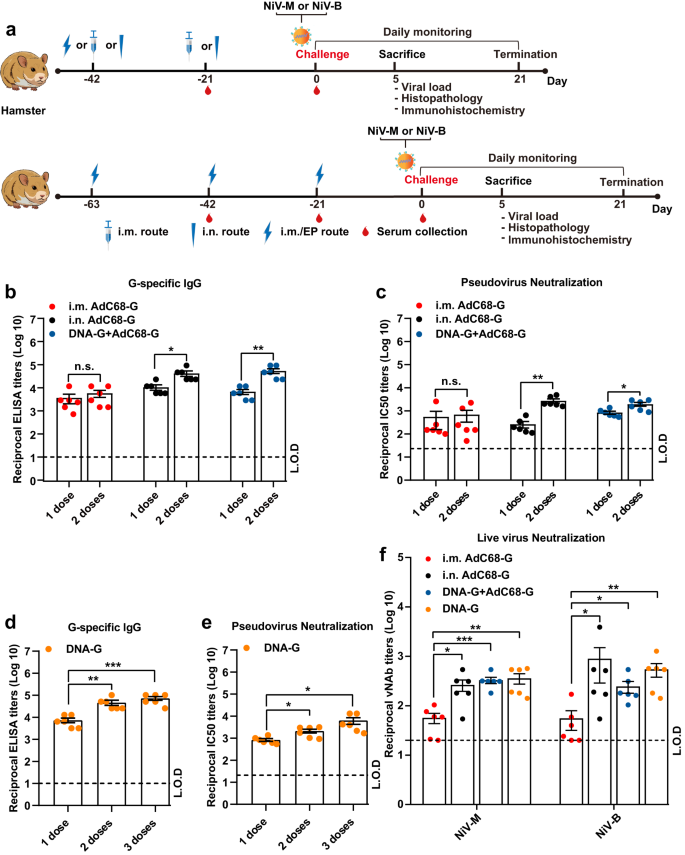
a The experimental scheme of Syrian hamsters. The animals were vaccinated at day 0 with AdC68-G (via the i.n. or i.m. route) or DNA-G (i.m./EP) and boosted with AdC68-G (via the i.n. or i.m. route) after 21 days (the upper) or immunized 3 times with DNA-G via the i.m./EP route (the lower) and then challenged with the NiV Malaysia and Bangladesh strains at 1000 LD50 via the i.p. route. Hamsters were monitored daily for survival as well as body weight changes. Six hamsters in each group (except for those in the AdC68-G (i.n.) group that was challenged with NiV-M, n = 3) were euthanized at 5 d.p.i. for viral load and pathogenesis analysis. The IgG antibodies (b) and pNAbs (c) on day 21 after the first or second vaccination. The IgG antibodies (d) and pNAbs (e) on day 21 after the first, second and third vaccinations. f vNAb detection based on the live NiV Malaysia and Bangladesh strains at 3 weeks after the last immunization. Data are presented as the group mean ± SEM. Statistical significance was determined one-way ANOVA with Tukey's multiple comparisons tests. n.s., not significant, *p < 0.05, **p < 0.01, ***p < 0.001. Error bars indicate 95% confidence intervals. L.O.D. represents the limit of detection.
Regarding the 2-dose groups, the results showed that after the first immunization, the AdC68-G and DNA-G regimens induced high levels of G-specific IgG and neutralizing antibodies, and the second vaccination resulted in a statistically significant increase (p < 0.05) in IgG (Fig. 3b) and pNAb (Fig. 3c) titers, except for in the AdC68-G (i.m.) group. For the 3-dose DNA-G vaccine group, G-specific IgG (Fig. 3d) and pNAbs (Fig. 3e) were detected in all hamsters, and pNAb titers increased (p < 0.05) following each immunization (Fig. 3e).
Hamster sera from both the AdC68-G and DNA vaccine regimen groups could neutralize two strains of NiV (Malaysia and Bangladesh), with the highest virus-neutralizing antibody (vNAb) titers of 567 (NiV-M) and 3903 (NiV-B), respectively. The vNAb titers in hamsters immunized with AdC68-G via the i.m. route was significantly lower (p < 0.05) than those in the hamsters in the other 3 groups, with two of the six animals in the AdC68-G (i.m.) group not having detectable neutralizing antibodies (vNAb titers <20). There was no significant difference in the neutralizing abilities against the NiV Malaysia and Bangladesh strains among two-dose AdC68-G (i.n.), DNA-G prime/AdC68-G boost and DNA-vaccinated animals (Fig. 3f). No IgG or neutralizing antibodies were detected in any of the control animals. These results indicated that the AdC68-G and DNA-G vaccines induced a potent humoral immune response in Syrian golden hamsters.
AdC68-G and DNA-G vaccines completely protected Syrian golden hamsters from lethal NiV infection
To confirm whether the vaccine regimen-induced immune response could provide protection against NiV challenge, all vaccine-immunized hamsters were challenged with a lethal dose of NiV (1000 LD50) via the intraperitoneal (i.p.) route 3 weeks after the last immunization (Fig. 3a). Hamsters (n = 3 per control group, n = 6 per vaccine group) were monitored daily for survival and body weight changes over a 21-day period, and six hamsters in each group were sacrificed at 5 days post-infection (d.p.i.) for viral load determination as well as pathogenesis analysis. During the monitoring stage, all the hamsters in the vaccine groups survived the lethal challenge, with no obvious signs of disease, and maintained a relatively stable body weight after NiV-M (Fig. 4a–f) and NiV-B infection (Supplementary Fig. 2a–f). In contrast, animals in the control group succumbed to the infection between 6 and 8 d.p.i. for NiV-M infection (Fig. 4a–c) and between 6 and 14 d.p.i. for NiV-B infection. Unfortunately, one hamster survived NiV-B infection in each control group, including the AdC68 (i.m. or i.n.) and pVAX1 prime/AdC68 boost groups, but there was a significant reduction in body weight (P < 0.05) even though euthanasia was not necessary (Supplementary Fig. 2a, b, d, e). The hallmark symptoms of disease, such as ruffled fur, slowed activity, hunched posture, bleeding, and labored breathing, were observed in the animals of the control group. The vaccine regimens completely prevented these symptoms. The clinical scores of the challenged hamsters were recorded (Supplementary Fig. 3). Statistical analysis demonstrated that survival in all vaccinated groups was significantly better than that in the corresponding control groups (p < 0.05).
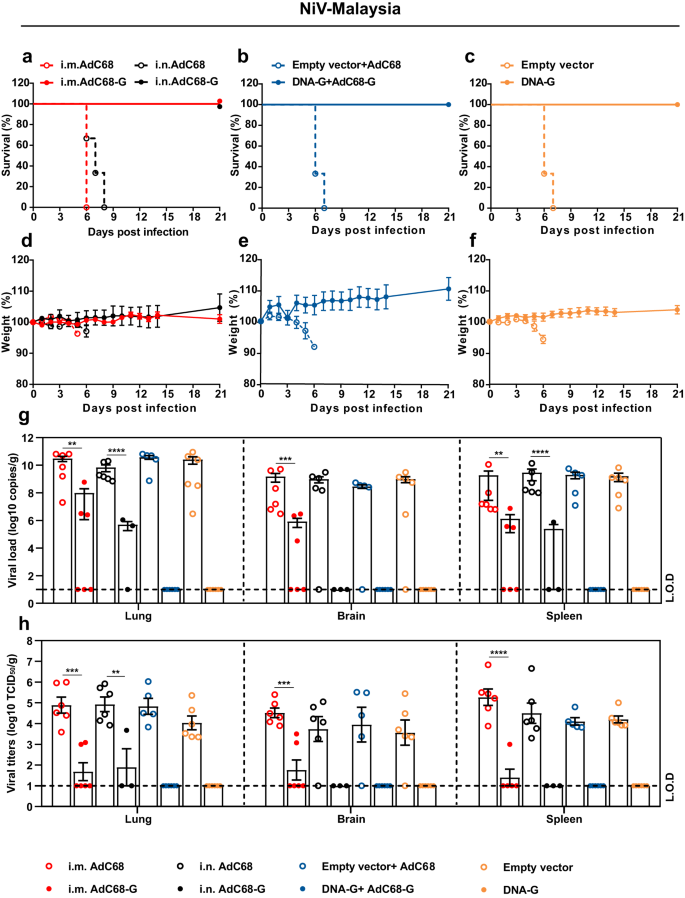
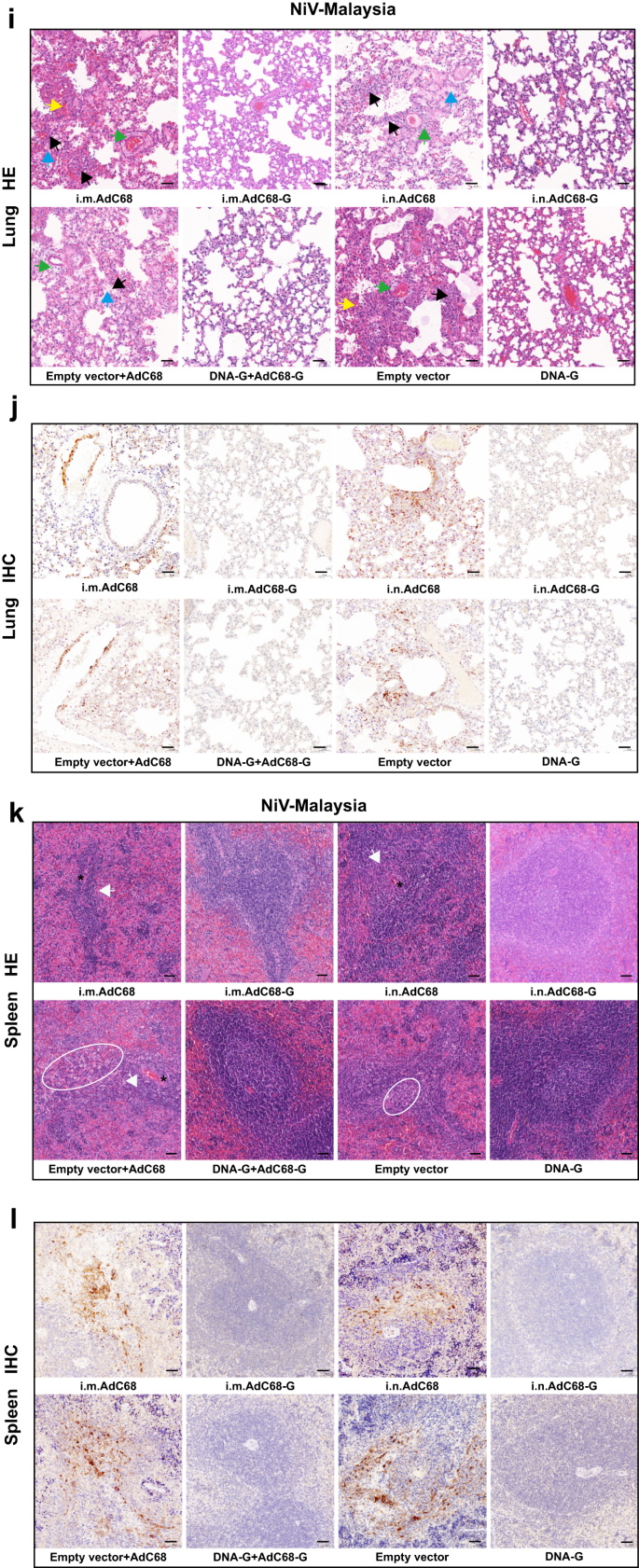
Survival (a–c) and weight change (d–f) of Syrian hamsters challenged with the NiV Malaysia strain. Viral loads in the hamster lungs, brain, and spleen at 5 d.p.i. quantified by quantitative PCR with reverse transcription (qRT–PCR) (g) and live virus titration (h). i Lung tissue sections were stained with hematoxylin and eosin (HE). Marked bronchointerstitial pneumonia with inflammatory cell infiltration or necrosis (black arrows), hemorrhage (yellow arrows), vasculitis (green arrows), and fibrous exudation (blue arrows) in the lung tissue of control animals. No pathology was observed in vaccinated animals. j Lung tissue sections were stained with an antibody against the NiV N antigen, which was visible as red‒brown staining (IHC). No immunoreactivity was found in vaccinated animals, whereas multifocal immunoreactivity could be found in the lung tissue of control animals. k Spleen tissue sections were stained with hematoxylin and eosin (HE). Loss of normal splenic architecture, with lymphocyte necrosis (white circles) and decreased lymphocyte numbers in white pulp (white arrows); the asterisk indicates the central artery. l Spleen tissue sections were stained with an antibody against the NiV N antigen, and red‒brown color indicates immunolabeled mononuclear or multinucleated cells. Data are presented as the group mean ± SEM. Two-tailed unpaired Student's t tests were conducted to compare differences between two experimental groups. **p < 0.01, ***p < 0.001, ****p < 0.0001. Error bars indicate 95% confidence intervals. L.O.D. represents the limit of detection. Bar scale, 50 µm.
Next, six hamsters in each group were euthanized at 5 d.p.i. to determine the viral load as well as the pathological changes in the lungs, brain and spleen. NiV RNA could not be detected in any of the three organs of the animals in the DNA-G and DNA-G prime/AdC68-G boost vaccine groups after NiV-M (Fig. 4g) or NiV-B (Supplementary Fig. 2g) challenge. For individual animals immunized with AdC68-G via the i.m. or i.n. route, NiV RNA was detectable, but the copy number of viral RNA was significantly lower (p < 0.01) in the tissues than those in the control group after challenge with NiV-M (Fig. 4g). The infectious particles were also examined in the tissues and showed the same pattern as viral RNA (Fig. 4h). In the NiV-B challenge experiment, all the animals in the vaccine groups except for those that received the AdC68-G vaccine via the i.m. route vaccination showed complete viral RNA elimination (Supplementary Fig. 2g). Infectious particles could not be detected in any tissues in any of the vaccine-immunized animals (Supplementary Fig. 2h). Pathological analysis of lung and spleen tissue collected at 5 d.p.i. revealed that the vaccinated hamsters maintained normal tissue structures with no obvious pathological damage after NiV-M (Fig. 4i, k) or NiV-B infection (Supplementary Fig. 2i, k), and no viral antigen was detected in lung and spleen tissues by immunohistochemistry (IHC) labeling for NiV nucleoprotein (N) antigen after NiV-M (Fig. 4j, l) and NiV-B (Supplementary Fig. 2j) infection. In the control group, decreases in lymphocyte levels and the loss of normal structures were observed in the spleen. Alveolar spaces had hemorrhage, edema and fibrin deposition with inflammatory cells, and inflammation often surrounded vascular and alveolar walls, consistent with a previous report (Fig. 4i, k; Supplementary Fig. 2i, k). In addition, NiV N antigen was detected by IHC in mononuclear cells, multinucleated cells, and the endothelium within the spleen and lungs (Fig. 4j, l; Supplementary Fig. 2j). Thus, these results indicated that the vaccines almost completely abolished viral replication and significantly alleviated the severity of lung and spleen pathology, ultimately providing complete protection against lethal NiV challenge.
Discussion
NiV is a re-emerging zoonotic pathogen that continues to pose a threat to human populations. Due to its high lethality, its potential for spread and the lack of effective medical countermeasures, the WHO has designated NiV as a priority pathogen requiring further research. Several NiV vaccine candidates have been tested in preclinical trials, including nucleic acid vaccines (DNA and mRNA)28,33,34, viral vector vaccines26,35,36,37,38,39 and subunit vaccines40,41,42. These vaccine candidates yielded different levels of protection against NiV infection; however, no licensed vaccines are available for NiV. Therefore, it is necessary to develop additional novel effective NiV vaccines to induce a potent and long-lasting antibody response and protect against a wide range of strains.
Genotypic analysis divides NiV into two strains: NiV-Malaysia (NiV-M) and NiV-Bangladesh (NiV-B)14,15. In our study, we aimed to optimize the immunogenic G sequence and develop a vaccine that protects against both strains using replication-deficient chimpanzee adenoviruses and DNA vaccines expressing full-length G protein. The results showed that a prime-boost approach using either AdC68-G or DNA-G induced a robust and sustained neutralizing antibody and cellular immune response in mice. Although the neutralizing activity of mice sera was detected by pseudovirus neutralization assay based on HIV backbone, the results were convincing, based on the results of others study33 and the results of the preliminary experiment (the correlation of live NiV neutralization and pseudovirus neutralization assays) of this study (Supplementary Fig. 4).
The heterologous DNA-G/AdC68-G immunization regimen produced higher T-cell responses and neutralizing antibody titers than homologous AdC68-G immunization. Furthermore, due to the coronavirus disease 2019 (COVID-19) pandemic, the heterologous severe acute respiratory syndrome coronavirus 2 (SARS-CoV-2) vaccine combination is gaining support for improving immunogenicity compared to that with the homologous vaccine administration used in mass vaccination campaigns for humans43,44. Though the AdC68-G vaccine of the study is a replication-deficient virus, it can still induce a durable (68 weeks) immune response in mice, which is consistent with other study30. While the exact mechanism behind the long-lasting and sustained immune responses is currently unknown31. The development of vaccines that induce a lasting immune response against respiratory viruses is currently the most challenging study. To fight against COVID-19, the vaccinees were recommended to be vaccinated every six months. Similarly, the seasonal influenza virus vaccine needs to be administered annually. All these are related to the difficulty of inducing durable immune responses in large animal models by the vaccines. In this study, the AdC68-G vaccine developed against Nipah virus induced an immune response lasting up to 68 weeks without a downtrend, which provides a promising and long-lasting vaccine against respiratory virus infection after being verified in large animal models such as African green monkey.
In human clinical trials, DNA vaccines for a variety of infectious pathogens yielded disappointing results45. There are lots of reasons for this poor performance, but a major reason is thought to be insufficient antigenic load due to inefficient expression of the target antigenic proteins in immunologically relevant cells and tissues46. To overcome the inefficient expression of the target antigen, EP is one of the most effective solutions. Histological analysis of the site of injection revealed that the transfection efficiency was greatly improved and the variability between animals was reduced when the DNA vaccines were delivered by EP. The advantage of in vivo EP is that it does work in larger animals, including humans47. Based on these, the delivery of the DNA vaccine in this study was performed by i.m/EP. Consistent with another research study48, the humoral and cellular immune responses induced by the DNA vaccine (delivered by EP) are not lower than or even higher than those of the protein vaccine under adjuvant.
Several immunization regimens with both vaccines provide complete protection against NiV infection. The heterologous DNA-G/AdC68-G and three-dose DNA immunization regimens were found to be superior to the homologous AdC68-G regimen. In the current study, we used the standard three-dose immunization regimen for the DNA vaccine. However, our immunogenicity data clearly show that a two-dose immunization regimen can induce a robust humoral immune response, and whether it can fully protect against NiV infection needs to be investigated further.
For the lethal NiV-B challenge, the infectious particles were eliminated in all vaccinated animals, while after the NiV-M challenge, no or reduced numbers of infectious particles were detected in the lungs, brain, and spleen in the i.n. and i.m. vaccinated groups. It is possible that our optimized G amino acid sequence had higher homology to that of NiV-B than to that of NiV-M. The Bangladesh strain shares 99.3% identity, while the Malaysia strain shares 96% identity. Notably, animals were given a high dose of the virus (1000 LD50) for the challenge in the current model. This infection dose is probably higher than that in a natural infection in humans.
In an outbreak scenario, an ideal vaccine should have the ability to elicit protective immunity after a single immunization dose. According to previous research, the developed adenovirus vector vaccine is capable of protecting against a virus challenge after a single immunization dose32,49. Thus, we investigated whether a single dose of AdC68-G (delivered by the i.m. or i.n. route) was sufficient to elicit an effective immune response in this study. The data presented here show that the prime-only approach induced robust T-cell responses and high levels of long-lasting neutralizing antibodies in BALB/c mice. The i.m. administration of AdC68-G elicited a significantly higher level of IFN-γ than i.n. AdC68-G administration. However, i.n. AdC68-G immunization appears to be more effective than i.m. immunization at inducing serum IgA immune responses. Whether the induced IgA can play the role of antibody-dependent cell-mediated cytotoxicity and then destroy the target cells infected by the NiV needs further study. Based on the results presented here, further studies are planned to test whether a single vaccination with AdC68-G can also protect animals against NiV challenge.
In conclusion, our results suggest that both homologous AdC68-G (delivered by i.m. or i.n. administration), heterologous DNA-G/AdC68-G prime/boost, and DNA-G three-dose approaches are effective in inducing immunity against Nipah virus infection in animal models. The heterologous DNA-G/AdC68-G prime/boost approach induced higher T-cell responses and neutralizing antibody levels, while a single dose of AdC68-G was sufficient to elicit a strong immune response in mice. Further studies are needed to determine if these findings can be applied to humans.
Methods
Ethics statement
The animal experiments were approved by the Animal Ethics Committee of the Wuhan Institute of Virology, Chinese Academy of Sciences (approval number: WIVA21202104). All animal experiments involving NiV were executed in the animal biosafety level 4 (ABSL-4) facility at the National Biosafety Laboratory (Wuhan), Chinese Academy of Sciences.
Cells and virus
HEK293T (ATCC: ACS-4500), HEK293 (ATCC: CRL-1573) and Vero E6 (ATCC: CRL-1586) cells were maintained in Dulbecco's modified Eagle's medium (DMEM, Gibco) containing 10% Fetal bovine serum (FBS, Gibco), penicillin (100 units/ml) and streptomycin (100 μg/ml) at 37 °C in 5% CO2. 293 Freestyle (293 F) cells were maintained in FreeStyle293 expression medium containing penicillin (100 units/ml) and streptomycin (100 μg/ml) in shaker incubators at 150 rpm, 37 °C, 8% CO2. The NiV Malaysia (AF212302.2) and NiV Bangladesh (AY988601.1) strains used in the challenge studies were obtained from the National Virus Resource Center, Wuhan Institute of Virology, Chinese Academy of Sciences. The virus stock was propagated in Vero E6 cells.
AdC68-Based NiV vaccine production
The full-length G sequences used in this study were obtained by optimizing the NiV G sequences of two different NiV-M and NiV-B strains in the software Geneious using the principle of sequence homology. Specifically, all the sequences of G proteins were downloaded from GenBank and the vaccine consensus sequences were acquired according to the sequence consistency principle. To understand the conservation of the acquired consensus sequence, a phylogenetic tree was constructed by the maximum likelihood method in Mega11. The G sequence was then codon optimized for humans and synthesized by GenScript (Nanjing, China). The codon optimized G gene was cloned into a transgene expression plasmid pShuttle2 between the restriction sites NotI and KpnI. Subsequently, after digestion with I-CeuI and PI-SceI restriction enzymes, the whole G expression cassette containing the CMV promoter, G gene, and BGH polyA tail was inserted into the E1-deleted region of the chimpanzee adenoviral vector pAdC68 to generate the recombinant pAdC68-G. The resultant pAdC68-G was linearized by PacI and transfected into HEK293 cells to rescue AdC68-G, Finally, AdC68-G as well as empty control AdC68 were propagated and purified by cesium chloride density gradient centrifugation, titrated, and stored at −80 °C.
NiV DNA vaccine construction
For the construction of the NiV DNA vaccine (also termed DNA-G), The optimized gene was subcloned into the clinically used vector pVAX1 between the restriction sites KpnI and BamHI with the Kozak sequence incorporated at the 5' end of the genes. The plasmid was transformed into E. Coli Top 10 cells for plasmid amplification and purified using an endotoxin-free Plasmid Plus Maxi Kit (Qiagen).
Western blot analysis
HEK293T cells cultured in 6-well plates were transfected with 2 μg of DNA-G or pVAX1 for 48 h. HEK293 cells were infected with AdC68-G and AdC68 at doses of 109 VPs per well. After transfection or infection, the culture supernatant was discarded, and the cells were harvested and lysed with 150 μl of RIPA lysis buffer containing a protease inhibitor cocktail. The samples were run on a 10% PAGE gel and transferred to a PVDF membrane (Millipore). After that, skim milk (5%) was added to block the membrane for about 1 h, and a mouse antibody against NiV-G serum (prepared by our laboratory) was used to incubate the membrane with a 1:500 dilutions. After washes, a horseradish peroxidase (HRP)-conjugated secondary anti-mice IgG (Proteintech, Cat No. SA00001-1, diluted 1:2000) was used to bind to the primary antibody. Finally, the membranes were developed with an ECL substrate (Thermo Scientific). The expression of GAPDH (Abcam, Cat No. ab8245, diluted 1:5000) was used as a loading control. Uncropped and unprocessed scans of are unloaded in the Supplementary Fig. 5.
Animal experiments
BALB/c mice (6–8 weeks old, female) and Syrian hamsters (5–6 weeks old, female) were purchased from Vital River Laboratories (Beijing, China). BALB/c mice were randomly allocated into 10 groups (Fig. 2b). In batch 1 (groups 1–4), two groups (groups 1 and 3) of mice were vaccinated (one or two doses) with 5 × 1010 VPs AdC68 via the i.n. route and were used as controls. Two groups (groups 2 and 4) of mice were vaccinated (one or two doses) with 5 × 1010 VPs AdC68-G by i.n. route. In batch 2 (groups 5–10), three groups (groups 5, 7 and 9) of mice were vaccinated with 5 × 1010 VPs AdC68 (i.m.) or 50 μg pVAX1 (i.m./EP) at day 0 and AdC68 (i.m.) at day 21 and were used as controls. Three groups (groups 6, 8 and 10) of mice were vaccinated with 5 × 1010 VPs AdC68-G (i.m.) or 50 μg DNA-G (i.m./EP) and AdC68-G (i.m.) at day 21. For DNA vaccine immunization in BALB/c mice, anesthetized mice were injected with 50 μl of solution containing indicated DNA, following instillation, the injection site was subject to electroporation at 100 V constant voltage with 3 pulses at 50 msec/pulse and 1 s intervals between pulses. Electric pulses were delivered by the electric pulse generator (ECM830; BTX, San Diego, CA). At day 10 after each vaccination, mice were s...
Comments
Post a Comment